
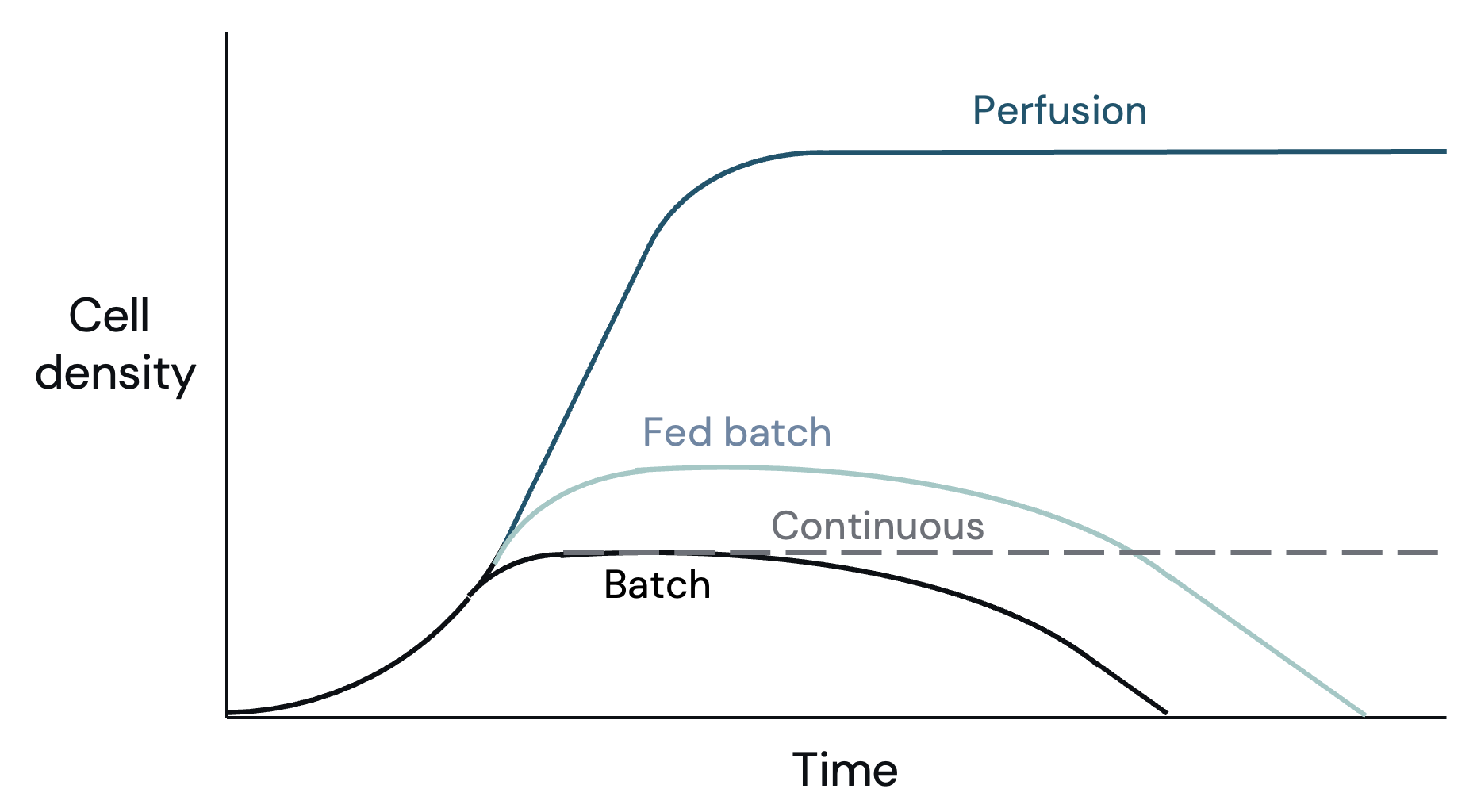
Cultivated meat’s path to parity: a techno-economic analysis
Using TEA, Yossi Quint, CEO & Founder of Ark Biotech, explains how cultivated meat could quickly become competitively priced alongside traditional animal-based meat
The cultivated meat industry is at an inflection point, with the conversation shifting from whether we can grow meat outside an animal (we can!) to unit economics and scale-up.
Achievements from industrial cell-culture in the pharmaceutical sector can serve as a baseline for an achievable cost structure for cultivated meat. Using pharmaceutical achievements as a baseline translates to cost of goods sold (COGS) of US$29.5/lb (excluding downstream, packaging, and food-service margins), reaching a cost comparable to filet mignon. There is ample room to further improve COGS, such as scaling-up media production and using larger bioreactors, as well as surpassing pharmaceutical achievements through innovation.
For cultivated meat to compete with traditional meat, COGS and CapEx need to decrease. Specifically, there are four major areas for cost-reduction:
Reducing the cost of media: The nutrients that feed the cells and spur growth are the single-largest operating cost. Decreasing the cost of inputs directly reduces COGS.
Improving cell mass: The cell characteristics play a defining role in developing a bioprocess. Using cells that can achieve higher densities or require less costly nutrients to grow means more mass with less input in less time, which improves COGS. Inducing differentiation can result in meaningful increases in mass.
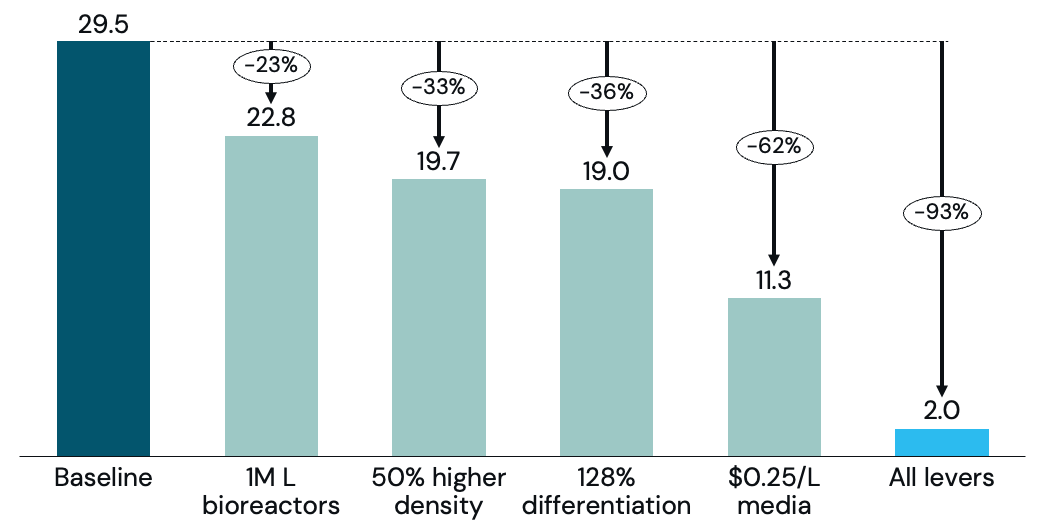
Optimizing the bioprocess: The mode in which nutrients are supplied to the cells (e.g. batch, perfusion, etc) impacts media productivity and bioreactor productivity. Selecting the bioprocess that is cost-minimizing is an optimization function that accounts for media costs, cell characteristics, and capital cost.
Reducing capital spend: Building bioreactors +10 times larger than the biggest bioreactor for animal cell-culture in use today (~25,000 liter) is the most effective lever to reduce CapEx, as bigger bioreactors cost less per liter of capacity and require less supporting equipment.
In this TEA, we have modeled the impact that each of the cost-reduction levers can have on further reducing COGS, and show that to reach price parity or superiority, cultivated meat will need to make progress across multiple levers (Figure 1).
Legacy cell manufacturing as a baseline
The pharma industry has been growing animal cells for decades. If cultivated meat were to be grown at industrial pharma-scale and achieve best-in-class pharma yields, upstream COGS would be US$29.5/lb (Figure 2), serving as a conservative baseline for cultivated meat production.
Although the COGS achieved in this industry can give us an example of where cultivated meat can land, animal protein and pharma end products entail very different pricing, margin, and demand-volume profiles.
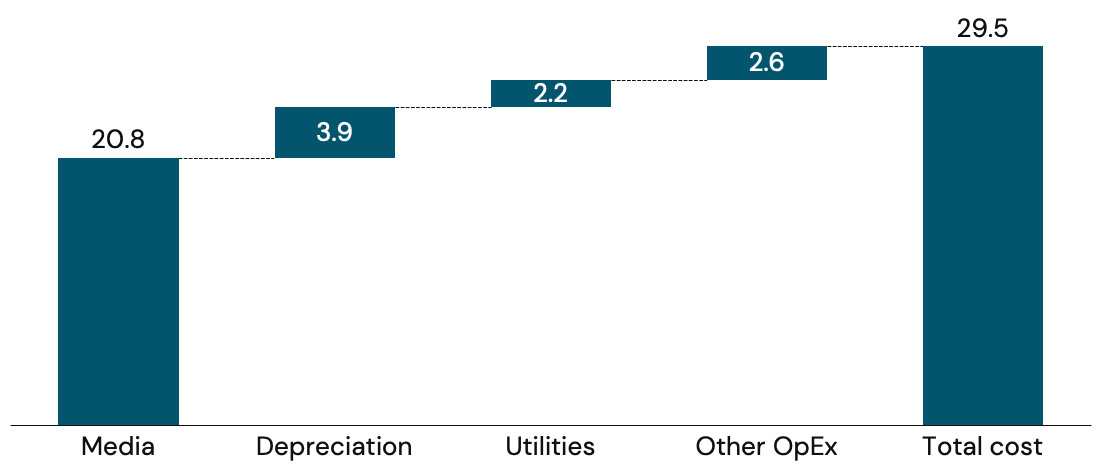
Pharmaceutical cell-culture manufacturers are optimizing for non-cost factors in production, and increasing production scale is not a pressing concern or even a desire.
A very large pharmaceutical factory may have 150,000 liters of bioreactor capacity, which is only enough to produce enough cultivated meat to feed a few thousand people each year.
Unlock 1: Media
Media is the key area where the baseline COGS of US$29.5/lb can be improved. Media costs can be reduced by firstly decreasing media production costs (e.g. procurement, recipe), and secondly increasing the cell mass per unit of media (growing more meat with the same amount of media).
The cultivated meat industry has made massive progress in media cost reduction, a process that is difficult, time consuming, and requires technological advances for step-change improvements. Yet, companies have made tremendous progress, including publicly shared milestones, such as US$2-per-liter media.
There are a number of ways that media costs can be further reduced:
Fit-for-purpose recipes: Growth per unit of media can be maximized by optimizing media ingredients and feeding strategy. For example, a cell may have the exact same growth if it receives 0.9 units of ingredient A versus 1.0 unit.
One-for-one ingredient replacements: Some traditional, and more expensive, media ingredients can be replaced with a cheaper analog that achieves the same function. For example, some companies have succeeded in replacing serum albumin with a chickpea-based ingredient.
Ingredients can also be optimized for other properties such as stability. Thermo-stable growth factors, for instance, have longer half lives, so can be used in lower concentrations.
Scaling up production of media ingredients: For many ingredients, especially those produced using a fermentation process, increasing the scale of production improves unit economics. Estimates from a media ingredient manufacturer suggest ingredient costs can be reduced 35-40% below pharma pricing just from increased scale with yield enhancements. Downstream process innovation could further reduce cost by ~50%.
Recycling media: Reusing parts of the media can also contribute to saving on media costs. Filtration or other bioseparation processes could be implemented to recover costly ingredients.

Unlock 2: Cell mass
The cultivated meat industry can also reduce the amount of media needed to produce a pound of meat by increasing biomass yield per liter of media. There are multiple ways to increase yield, including:
Optimize cell lines to achieve higher densities, naturally or through genetic modification: Optimization may be a multi-pronged process, such as increasing metabolic efficiency (e.g. reducing lactate/ammonia production, introducing new enzymatic pathways) or screening for cells with higher tolerance for fluctuations in certain culture conditions (e.g. pH, temperature, osmolarity).
Optimize for mass increase during differentiation: Differentiation increases the mass per cell but it also increases the time needed within a bioreactor. Although robust data on mass increase during differentiation is limited, studies have shown that mass per cell can increase 2.28-30 times differentiated over a three- to 20-day process. Differentiation can significantly reduce media needed per pound of production (Figure 3) and cost (Figure 4).
Remove constraints that are limiting cell growth, such as metabolic (e.g. lactate, ammonia) waste build-up: This can be accomplished through trusted methods like perfusion or more novel methods such as genetic modification of the cells.
Unlock 3: Bioprocess
Optimizing the bioprocess is essential to minimizing COGS and CapEx as the bioprocess impacts cell growth and media usage. Fed-batch and continuous processes are the optimal modes for most processes at scale. Fed-batch is often COGS minimizing, while continuous has lower CapEx requirements.
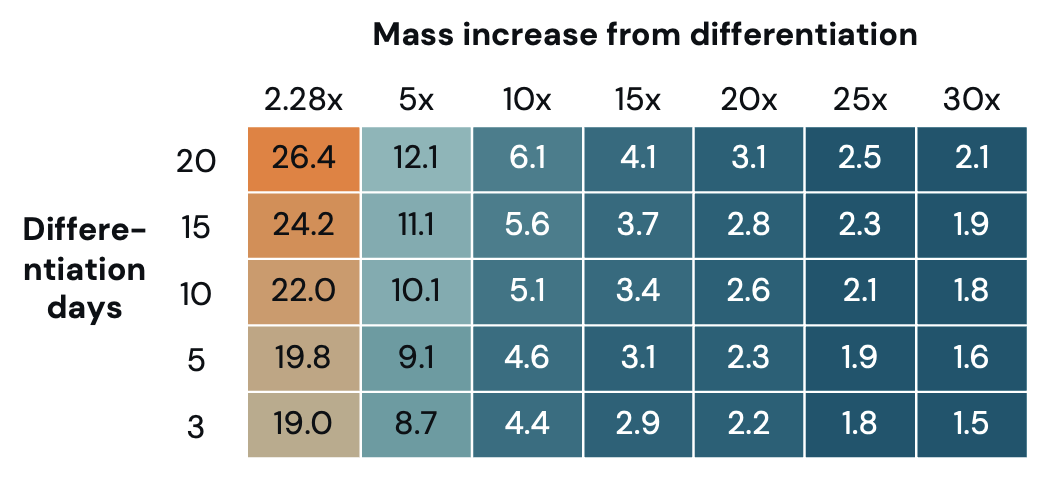
When comparing the cell density achieved by each process, perfusion clearly wins (Figure 5). However, the additional media needed to achieve those high densities means that perfusion does not have better media productivity (mass produced per unit of media) than a batch or continuous process (Figure 6). Fed-batch is the only process that by design has higher media productivity, requiring less media to achieve the same output – an advantage when media costs are high.
Cultivated meat COGS can be further reduced through blending cultivated meat with plant-based or fermentation-derived ingredients
Bioreactor modes
Each of the four modes involve trade-offs. The decision as to which mode to run is an optimization function based on cell lines, achievable yields, media cost, and operating constraints.
Batch: Cells grow in the initially supplied batch of media. The media begins with max nutrients, and these nutrients are consumed over time by the cells. Cell growth slows down and ends when there are not enough nutrients left or metabolic waste becomes too concentrated. Batch is the simplest process operationally. In the pharma industry, batch can achieve yields of ~12 million cells/mL.
Perfusion: Perfusion removes media from and adds media to the bioreactor, enabling cells to grow for longer and productivity per bioreactor to improve. A cell-separation device is used, so that the cells stay in the bioreactor even while media is removed. This process requires additional equipment and consumables, which are expensive and limited in scale. Cell density can reach +100 million cells/mL. However, as the perfusion process is consistently adding in new media, cell yield per unit of media does not increase.
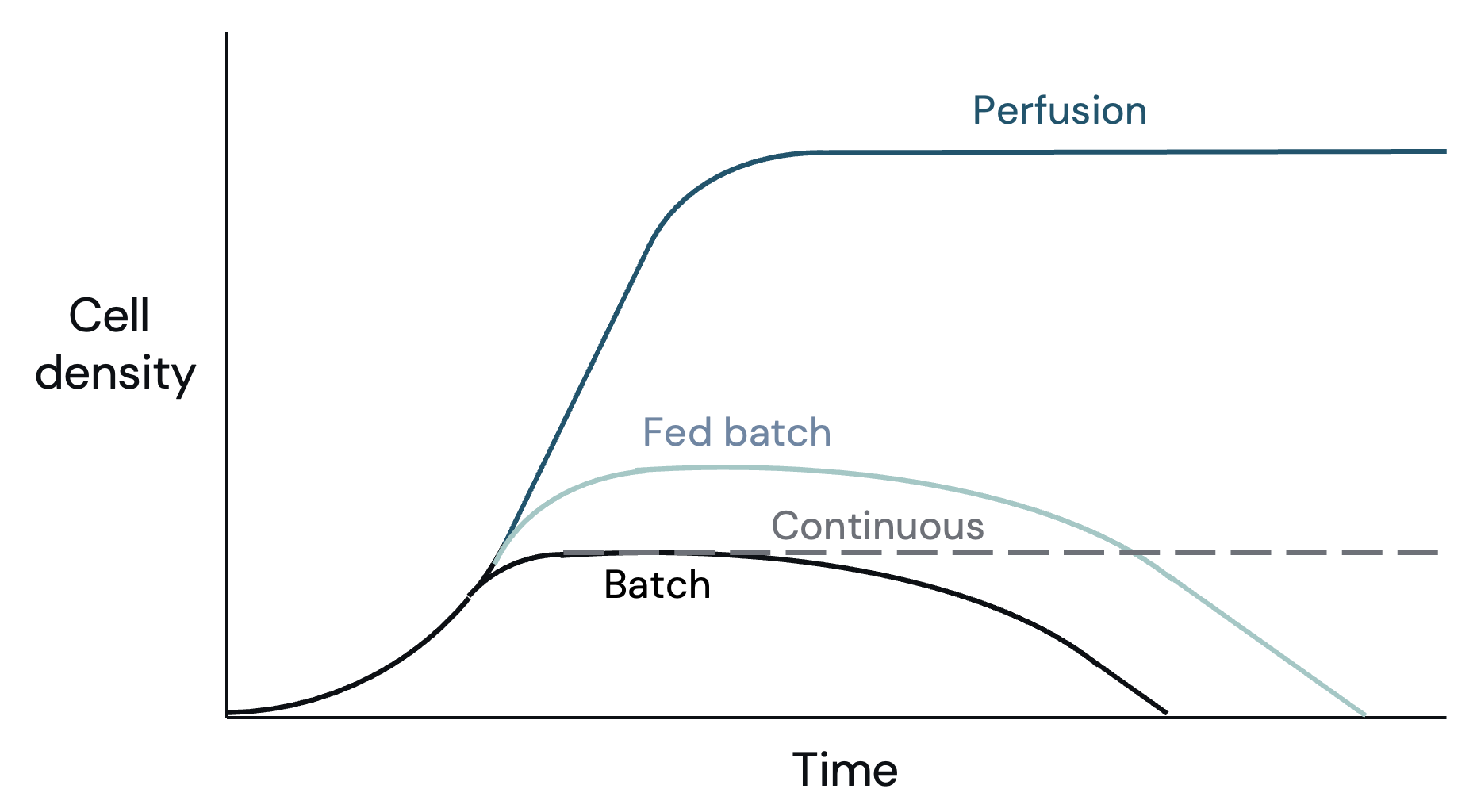
Fed-batch: The bioreactor starts partially filled with additional media added during the run. Adding new (more concentrated) media allows the cells to maintain their optimal nutrient consumption for longer, allowing cells to grow for longer and reach higher densities than in a batch process. In the pharmaceutical industry, fed-batch can achieve yields of ~20M cells/mL.
Continuous: Continuous (also known as bleed) removes media from and adds media to the bioreactor, enabling cells to grow for longer and productivity per bioreactor to improve. Continuous mode is more advantageous if the doubling-time is significantly shorter than 24 hours. Unlike perfusion, continuous removes media and the cells contained therein as there is no cell-separation device (no added equipment or consumable cost). There is no improvement in yield per unit of media.
Perfusion and continuous have one major advantage over fed-batch: bioreactor productivity. In most instances, continuous will be advantageous to perfusion as continuous has better bioreactor productivity, while maintaining similar media productivity.
Continuous production has another cost advantage in that there are limited additional equipment or consumables needed. The potential drawback of continuous is that the downstream needs to be able to support continuous or near-continuous harvesting.
Continuous processes require significantly less CapEx, hence lower depreciation, than fed-batch as fewer bioreactors are needed to achieve the same production output.
Conversely, fed-batch requires less media use as it yields more mass per liter of media.
Whether continuous or fed-batch is a superior process, therefore, comes down to cost of media and depreciation. Using bioreactor size as a proxy for capital cost, we compared continuous and fed-batch COGS for scenarios from US$2.00/liter to US$0.25/liter media costs and from 25,000 liter to one million liter bioreactors. In almost every scenario, fed-batch has lower COGS but higher CapEx driven by fed-batch’s lower bioreactor productivity.
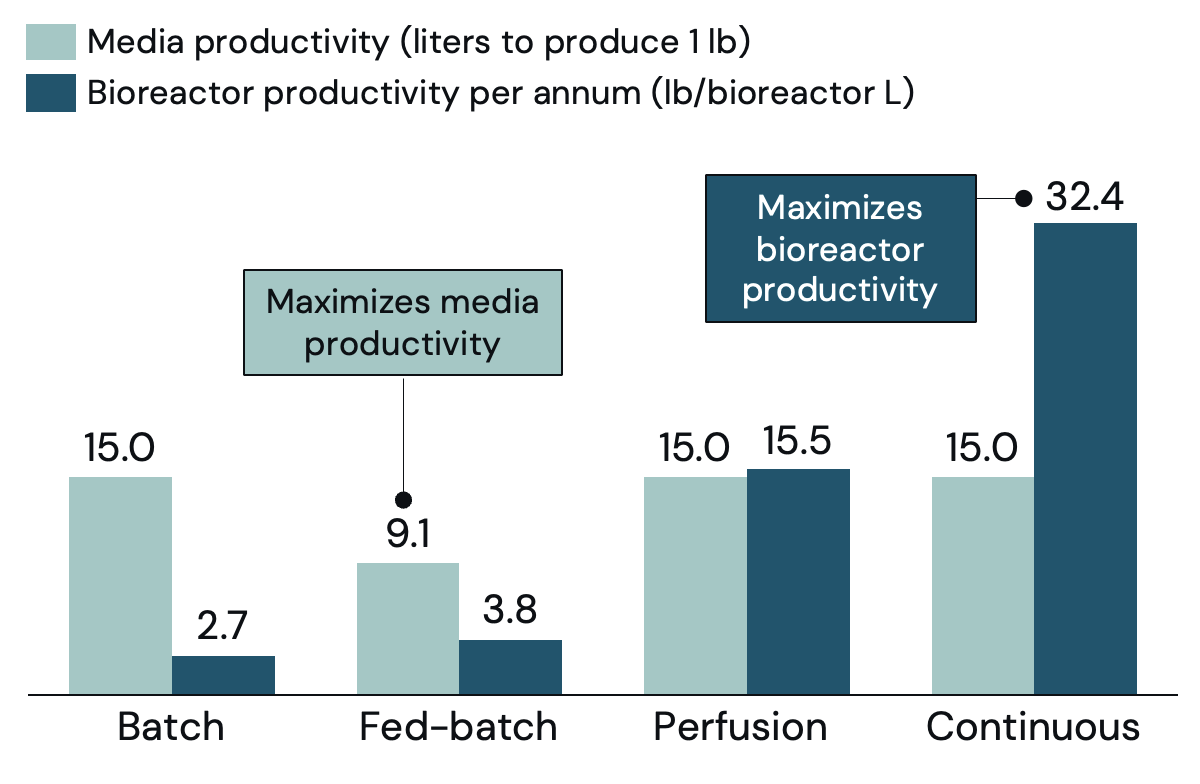
Unlock 4: Bioreactors
Within the factory, bioreactors are the most expensive piece of equipment. The single-biggest lever to reduce bioreactor cost is scale.
Reducing bioreactor spend is critical for reducing capital cost as bioreactors account for +25-50% of total upstream factory cost. A significant portion of bioreactor costs are fixed and most variable costs scale at a factor <1, so increasing scale reduces cost per liter. Increasing the bioreactor scale also reduces the number of pieces of external equipment needed per liter of production, further decreasing total CapEx per liter of production capacity.
Traditional stirred-tank bioreactors, however, are difficult to scale to hundreds of thousands of liters without degrading performance characteristics, so new technologies such as Ark’s bioreactor design, are needed to build commodity scale bioreactors. Today, the largest industrial bioreactors (almost all, if not all, of which are stirred tanks) have a working volume of about 25,000 liters. Stirred-tank bioreactors can be bigger than 25,000 liters, but cannot scale by an order of magnitude or more without sacrificing performance due to technological constraints and trade-offs, such as mixing, mass transfer, and shear.
Increasing scale by an order of magnitude could reduce total upstream depreciation per pound by 65% with additional savings available at even larger sizes (Figure 7).
CapEx costs can be further reduced through creating fit-for-purpose bioreactors. A cultivated meat specification could have reduced sterility requirements, reduced pressure vessel requirements, and leverage lower-priced materials.
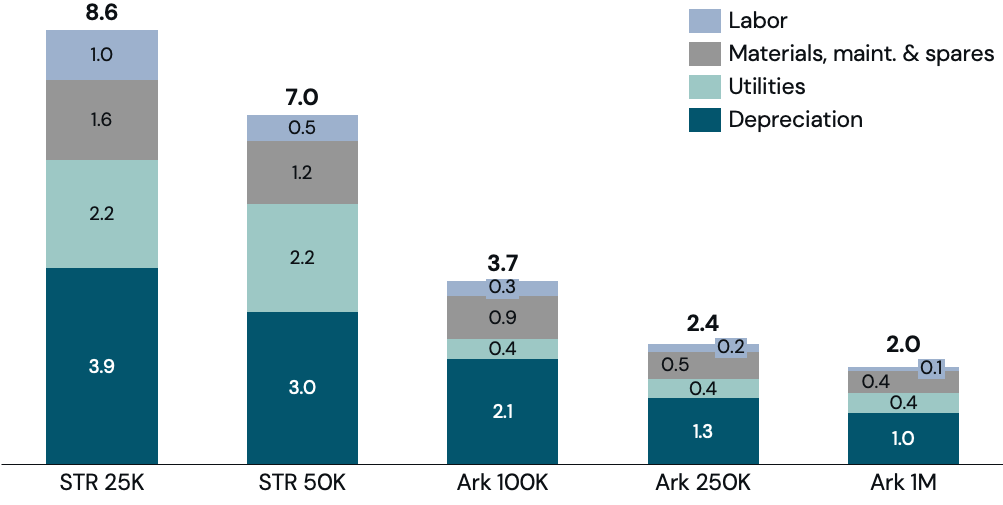
The path forward
There are multiple ways cultivated meat can achieve price parity or superiority relative to conventional meat. At the very least, media costs will need to be reduced, cell growth will need to reach higher densities, bioprocess will need to be optimized, and equipment spend will need to come down. Cultivated meat COGS can be reduced more by blending cultivated meat with plant-based or fermentation-derived ingredients.
A great deal of innovation has been made across the cultivated meat ecosystem. With continued focused innovation, especially in the four areas highlighted in this TEA, cultivated meat can achieve price parity. With so much at stake, there’s no time to lose!
For more information about Ark Biotech, please click here
If you have any questions or would like to get in touch with us, please email info@futureofproteinproduction.com